Earthquake school in the cloud: Citizen seismologists in Taiwan
This is an article accepted in Seismological Research Letters (Columns: EduQuakes).
Authors:
Wen-Tzong Liang1, Kate Huihsuan Chen*2, Yu-Feng Wu3, Eric Yen4, Chun-Yen Chang3
1. Institute of Earth Sciences, Academia Sinica
2. Department of Earth Sciences, National Taiwan Normal University
3. Science Education Center, National Taiwan Normal University
4. Grid Computing Centre, Academia Sinica
(somehow the figures are not shown properly on this webpage, please request for pdf file if needed)
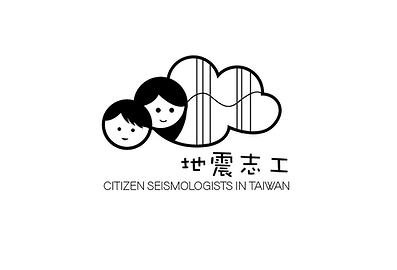

Figure 1. (a) QCN and (b) P-alert citizen seismic networks in Taiwan. Grey area represents higher ground with elevation higher than 500 m.
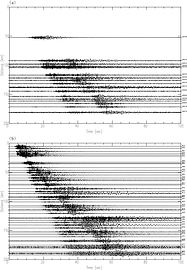
Figure 2. Waveforms recorded by (a) QCN-Taiwan network and (b) P-alert network for the 2013/10/31 Mw 6.3 Ruisui Earthquake that occurred in Hualien, Taiwan. Only one trace is plotted within each 4 km distance interval to allow the P-alert waveforms to be viewed clearly.
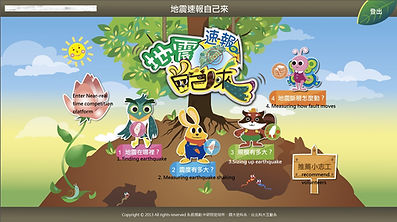
Figure 3. The front page of the near-real time earthquake games competition website. Each animal represents a different game. Once the certificate is achieved, the user can enter the competition platform (indicated by the flower) to contribute to real earthquake information.
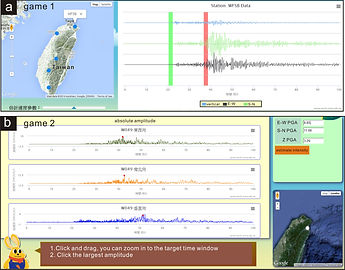
Figure 4. Waveform example for the four CSTaiwan certificates: (a) Finding earthquake by picking the first arrival of P and S waves. (b) Measuring earthquake shaking by clicking the largest amplitude in three components of seismograms.

Figure 5. Waveform example for the four CSTaiwan certificates: (a) Sizing up an earthquake by clicking the largest amplitude in the horizontal component of a seismogram. (b) Measuring how a fault moves by deciding whether the first P wave motion is up or down.

Figure 6. Epicentral distance as a function of travel time difference (S wave arrival minus P wave arrival) using a collection of earthquakes in Taiwan.

Figure 7. Visual comparison in lower-hemisphere project between (a) the observed initial motion recorded by various seismic stations processed by the user and (b) the sketch of fault plane solutions for normal, thrust, left-lateral, and right-lateral faulting.
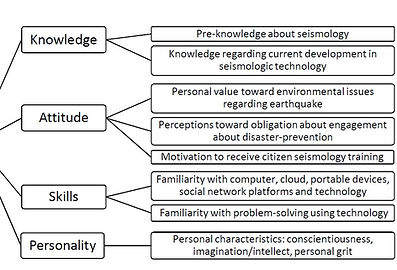
Figure 8. The proposed model of Citizen Seismologic Literacy (CSL) with associated detailed dimensions.

Acknowledgement
We thank Associate Editor Alan Kafka and anomalous reviewer for their invaluable suggestions and comments. We appreciate Yih-Min Wu who has shared the real-time data from Taiwan early-warning system (Palert) for the educational purpose of this project. Our deep gratitude goes to Shu-Jane Huang who handles the technical details of the earthquake game platform and Ting-Chen Yeh for the kind assistance. We also thank the visualization design team led by Ryan Wang and Hsin-Hui Huang in Dep. of Interaction Design in National Taipei Univ. of Technology. The seed teachers in our working group are also greatly appreciated for their feedback and development on teaching materials. The project is supported in part by the Ministry of Science and Technology, Taiwan (previously named National Science Foundation of Taiwan) under the Grant number MOST 103-2116-M-001-018 and 102-2511-S-003-052-MY3.
Introduction
To better prepare citizens for future impacts of natural disasters, it is important to understand how previous disasters have occurred, why lives were taken, and what lessons have been learned. Such understanding allows the attitude of the public to change from training to learning for natural disaster preparedness. Taiwan is one of the most vulnerable places on Earth for natural hazards, with 73% of its land area and population exposed to more than three types of natural hazards (Dilley, 2005). Unlike floods, landslides, and cyclones, earthquakes only afford a short period of time for reaction and response (e.g., usually less than 10 seconds). It is therefore crucial to increase the capability and knowledge of the Taiwanese citizens regarding earthquake science, specifically as to why earthquakes happen, how they occur, and how we should best prepare ourselves and the city for an earthquake hazard.
It is hoped that through the cultivation of a crew of volunteer citizen seismologists, public involvement could be encouraged and the discovery and inquiry into earthquake knowledge could be promoted. These volunteers can contribute to data collection, analysis, and reporting, and have the potential to greatly improve the emergency response to earthquakes. The Citizen Seismologists in Taiwan Project (CSTaiwan) is designed to elevate the quality of earthquake science education by incorporating earthquake/tsunami stories and educational earthquake games into traditional school curricula. The project aims to build a cloud-based computing service incorporating an earthquake school (i.e., a website for online learning) where teachers can easily teach their students about earthquakes and children can learn about earthquakes in a fun environment.
Through a pilot program of courses and professional development workshops, we have worked closely with teachers in elementary, junior high, and senior high schools, to design workable teaching plans incorporating the practical operation of seismic monitoring at homes and schools. As well as providing an update on the citizen seismic networks in Taiwan, this paper demonstrates how students perform P- and S-wave picking and measure seismic intensity through an interactive learning platform, how scientists and school teachers work together, and how we create an environment to facilitate continuous learning (via a near-real time earthquake games competition) while making earthquake science fun.
Citizen seismic networks in Taiwan
We have been collaborating with Stanford University to maintain a regional Quake-Catcher Network (QCN) server in Taiwan (Cochran et al., 2009) for promoting citizen seismology in Asia. Over the past two years, more than 170 volunteers in schools requested sensors and installed these sensors in Internet-enabled computers. System logs indicate that the sensors are on average active 50–60% of the time. This data set has been used for reporting seismic intensity and online waveform analysis. Figure 1a shows the current QCN site distribution in Taiwan, mainly covering the highly populated areas. To increase the spatial resolution of sensor data, we also implemented an online interface to receive real-time data from the existing P-alert strong motion network (Wu et al., 2013), which features up to 543 stations (Figire 1b) and availability of >80%. Figure 2 demonstrates the improvements in P-alert data coverage at various epicentral distances. The P-alert network is composed of low-cost MEMS accelerometers, which are embedded with real-time seismological algorithms to provide on-site earthquake early warning (EEW, Wu et al., 2013) as well as near real-time ground motion intensity measurements. Due to the success of the online earthquake early warning system (P-alert), both the Minister of Education and the Minister of Science and Technology have attempted to promote this island-wide school network.
The P-alert signal resolution is 16 bits with a -2g to +2g range and the sampling rate is 100 samples per second. The full-scale range of QCN MEMS sensors is also ±2g, but most of them are of 12 bits resolution. Only few 16-bit sensors were given to volunteers who are located in relatively higher seismicity area. The sampling rate of QCN waveform is 50 samples per second. In addition to the differences in sensor specification and embedded seismological algorithms, QCN sensors were all hosted by school volunteers, whereas the P-alert devices were installed mainly in schools and maintained by technicians of the P-alert team.
The recorded waveforms for QCN and P-alert for the 2013/10/31 Mw 6.3 Ruisui Earthquake that occurred in Hualien, Taiwan are shown in Figure 2. It is obvious that the quantity of recorded P-alert waveforms is much higher than those recorded by QCN-Taiwan. But the combined QCN and P-alert citizen seismic networks can provide dense station coverage and a high-resolution seismic intensity map. Continuous signals collected at volunteer homes/schools are streamed to Academia Sinica in Taiwan for data archiving.
However, there is often a demand for seismic data access and education from the volunteers, in particular the high school teachers. Due to this demand, we aim to not only encourage volunteers to install novel cheap sensors (~$70 USD) at homes and schools, but also to make the recorded seismic data useful in classrooms. To begin with, we developed teaching materials that enable citizens to learn the information hidden in seismograms: For example, where and how big the earthquake is, and what type of faulting occurred. These teaching materials are engaged in a near-real time earthquake games competition. Below we will introduce the teaching resources and games in Sections 3 and 4, respectively.
Teaching resources
To help volunteers understand the basic seismology/physics, we developed teaching materials that enable citizens to learn through a YouTube video clip (1-6 minute long), or enable teachers to teach in their classrooms. We prepared six teachable units closely tie in informative stories with the practice earthquake games (Section 4). Units consist of the following: (1) Orphan tsunami (story), (2) Finding earthquakes (game), (3) The 2004 Sumatra earthquake and tsunami (story), (4) Sizing up earthquakes (game), (5) Forensic seismology (story), and (6) Making fault motions (game). The learner can watch the YouTube video for pre-education purpose. In our pilot, each unit takes about 30-minutes in order to introduce the important concept.
Each “story” has an associated “game” unit. Unit 1 (Orphan tsunami) describes the real tsunami event that occurred on January 26, 1700 (Atwater et al., 2005). This mysterious tsunami arrived at Japan along the coastline from north to south over a distance of 1000 km, leading to flooded fields, wrecked houses, fire, shipwreck, evacuation, and panic. However, no earthquake was felt in Japan. The 1700 tsunami remained an orphan for ~300 years. In the 1980s, geologists found the remains of sunken marshes and forests as evidence of a "parent" earthquake in North America, in an area that was not known to have experienced an earthquake greater than Mw 7.5 in recorded times. This unit teaches (1) why a subduction zone earthquake is dangerous; (2) under which conditions, following the earthquake, the devastating tsunami was initiated; and (3) how to find evidence of past earthquake and tsunami events. In the modern world, however, earthquakes can be found by reading seismograms. This leads to Unit 2, where students participate in an earthquake game called “Finding Earthquakes”. By picking P- and S-wave arrivals at more than three stations, the students are able to locate an earthquake. The teaching material and practical application teaches (1) what P-, S-, and surface waves are; (2) how to pick P- and S-waves; and (3) how to use the time difference between P and S arrivals to determine the earthquake location.
Unit 3 describes the Sumatra earthquake and tsunami. In 2004, a Mw9.3 earthquake occurred in northern Sumatra, the biggest event since the 1960 Mw9.5 Chile earthquake. The rupture propagated northward with a speed of ~2 km/s, covering 400 km within 200 seconds. On the surface, the strong shaking lasted 8 minutes. Fifteen minutes following the earthquake, a tsunami hit Aceh and caused ~100,000 deaths, at a location where people were not prepared for disastrous tsunami. The Pacific Tsunami Warning Center did in fact issue a warning tsunami bulletin 15 minutes after the occurrence of the earthquake, however the warning claimed that “no destructive tsunami threat exists” due to the underestimation of the earthquake magnitude as Mw8.0. Therefore, the quick and precise determination of earthquake magnitude is key to detecting tsunamis in advance and to having the ability to issue warnings to prevent loss of life. This unit teaches (1) what the precursory signals before a massive tsunami are; (2) how the amplitude of the seismic signal correlates with earthquake magnitude; and (3) what information is required for effective tsunami warning. This leads to Unit 4, the “Sizing Up Earthquake” game, where students can measure the magnitude of an earthquake by picking the maximum amplitude on three component seismograms.
Unit 5 (Forensic Seismology) aims to show how a seismologist acts as a forensic scientist to detect secret nuclear test explosions, mine collapses, and submarine explosions. This unit teaches (1) historical ground-shaking events that require seismologists to find the source; and (2) seismic discrimination between earthquakes and explosions/implosions. One of the seismic signatures that clearly shows the distinction between an earthquake and an explosion is the "focal mechanism", which describes which type of earth movement produces the earthquake. It refers to the orientation of the fault plane that slipped and is also called the fault-plane solution. This is introduced in the following Unit 6, the “Making Fault Motions” game. By picking the initial motion polarities of the first arrival of the P wave, the fault type (normal, thrust, or strike slip) can be determined.
Near-Real time Earthquake Game Competition
We also developed a near-real time earthquake games competition for all registered citizen seismologists in Taiwan, to engage the learner in earthquake sciences by playing with real seismic data. Volunteers are encouraged to install the QCN sensor at home or school and to register as a citizen seismologist. The competition platform allows citizen seismologists to report earthquake information by processing P- and S-wave arrivals (Finding Earthquakes game), peak ground motion (Measuring Earthquake Shaking and Sizing Up Earthquakes games), and first motion of P-waves (Measuring How a Fault Moves game) after 10 minutes of an earthquake event. The front page of the platform is shown in Figure 3. Citizen seismologist here are required to complete the four levels of CSTaiwan certificates: (1) Finding the earthquake – by picking P- and S-wave arrivals at more than 3 stations, the epicenter of the earthquake can be found (Figure 4a). (2) Measuring earthquake shaking – by picking the maximum amplitude in three components of seismograms, peak ground motion can be measured (Figure 4b). (3) Sizing up earthquake – by picking the maximum amplitude in the horizontal component with the previously defined epicenter, earthquake magnitude can be measured (Figure 5a). (4) Measuring how a fault moves – by picking initial motion polarities of P wave first arrival (up or down), the fault type (normal, thrust, or strike slip) can be determined (Figure 5b). Once the four certificates are achieved, the citizen seismologist can challenge themselves by taking part in the near-real time competition, where the same data processing skills are required.
The methods applied in the four games are detailed in the paper but not listed in this webpage.
Within 10 minutes of a M4 earthquake in Taiwan, the near-real time waveform data from citizen seismic networks are released and the competition begins. The citizen seismologists have three days to play with the real seismograms and compete with each other regarding the precision of the location of the epicenter, magnitude, and fault plane solution.Based on the scores, the top five players are announced and updated in real time. The users are encouraged to create their own earthquake history by saving the intensity map each time it is processed. Since September in 2014 when the near-real time competition was announced through outreach activities, the QCN volunteers increased by 45 and student volunteers increased by 163. To encourage more users to get familiar with this platform, we open guest accounts upon request. This is probably the main reason why the newly installed sensors are on average active ~50% until May 2015, almost the same compared to that prior to the release of game. The impact assessment on QCN practice/maintenance, change in knowledge, attitude, and skill in earthquake sciences will be further investigated in the near future.
Outreach and Citizen Seismology Literacy (CSL)
Since May 2013, a series of 3rd ~12th grade science teacher workshops have been conducted to enhance our educational materials for the purpose of outreach. We then applied the enhanced materials in several teacher professional development workshops across the nation. With 15~80 participants in each workshop, reaching hundreds of teachers of 1st ~ 12th education, we continually collect and analyze a variety of the participants’ demographical information, pre-knowledge of seismology, personality self-portraits, feedbacks toward workshops, and other related data for potential future directions of the project.
We developed 49 questions associated with participants’ pre-knowledge, attitude, and skills in earthquake sciences called Citizen Seismological Literacy (CSL), while their demographic information (e.g., gender, age, educator experience, etc.) and personality (i.e., personality self-portraits) were surveyed by 16 questions, as illustrated in Figure 8. Personality in the current study was studied by three sub-factors: (1) Conscientiousness (four questions), defined as a desire of personality to complete a task well, (2) Intellect/Imagination (four questions), defined as the ability of personality to understand or depict abstract ideas (Donnellan, Oswald, Baird, & Lucas, 2006), and (3) Grit (eight questions), defined as “perseverance and passion for long-term goals” (Duckworth, Peterson, Matthews, & Kelly, 2007, p. 1087). In sum, 65 questions were applied to study the proposed CSL model.
At the present stage, however, for the purpose of having the CSL model reach the citizen, in addition to the aforementioned in-services teachers, we also tested the model within a national normal university in northern Taiwan. As a result, a total of 121 pre- and in-service teacher participants’ data have been analyzed.
We assess whether personality self-portraits may have an effect on CSL (as shown in Table 1), where the asterisks indicate significant correlations. To illustrate, column 1 shows Knowledge is significantly correlated with Skill; Column 2, Attitude is significantly correlated with Skill and all Personality sub-factors; Column 3, Skill is significantly correlated with all Personality sub-factors; and in column 4, CSL serves as a summary of Knowledge, Attitude, and Skill (1+2+3), is significantly correlated with all Personality sub-factors. As a result, all three sub-factors in Personality are positively related to most CSL dimensions (e.g., Attitude and Skills, but not Knowledge), providing evidence that Conscientiousness, Intellect/imagination, and Grit have an effect on developing one’s seismology literacy (CSL).
The CSL model developed in this study may serve as an example to quantify citizen’s background in earthquake sciences. The factor controlling CSL is explored as well, which may provide a better approach to citizens’ learning paths for promoting citizen seismology in the future. The proposed CSL model could be applied as a framework for seismologists around the world who wish to approach the public for educational purposes, while considering promoting the public’s seismologic literacy.
Challenges and future plan
As primary and secondary educators were believed to be the best candidate seeders in the Taiwanese educational system because they have the potential to influence a large number of people through the student population. Thus, we particularly targeted this group and studied how they might interact and promote the idea of CSL via the QCN series workshops, and further utilize the materials for teaching. However, once the participating teachers activated the QCN service, most of them failed to keep their QCN sensors operating.
We found indications that this might be associated with the personality of the teachers (i.e., level of conscientiousness and grit), or the fact that the educational system does not facilitate educators’ imagination/intellect. However, the situation may have been more complex due to the high pressure associated with the annual high school entrance test, which determines the future of 200,000 pupils every year (Yeh et al., 2009; Bronson and Merryman, 2013). Teachers may also have found that supplemental teaching outside of their course materials increased their workload by an unreasonable amount. In this scenario, CSL could hardly be promoted.
Further feedback from teachers included their lack of confidence in teaching the science behind the series of QCN activities. This appeared as a common theme in our data, particularly among those teaching in elementary schools. From these results, we conclude that QCN installation and maintenance is not straightforward, observational seismology requires the skill to process the earthquake data, and there is a lack of sufficiently use-friendly, interactive tools for data sharing and networking. This represents a major challenge that the educational seismology community will need to address if operating seismographs in educational environments is to be a truly successful endeavor.
To motivate learning, users will need to have an understanding of how to read seismograms, what to see in the data, and why their contributions are important. This can now be achieved by an interactive tool, the Near-Real Time Earthquake Game Competition (qcntw.earth.sinica.edu.tw/games/competitionV2/). Through a series of pilot courses and development workshops, we found that the most efficient learning happens when the audience is engaged in a competitive environment without a priori introduction to seismological background. In this scenario, participants often asked for supporting materials to better understand the physics behind the earthquake game. This is consistent with our CSL investigation result that background knowledge is not the most important factor. Rather, the attitude and skills of participants (highly correlated with personal grit level) are most important.
The CSTaiwan website (katepili2003.wix.com/future-eq-school) provides the teaching resources, workshops announcements, links to access data feeds, and updated activities. Content is currently available in Chinese for Taiwanese citizens, while the English version of the competition game and teaching materials are still under development. In the future, we hope to collaborate with other citizen seismic networks from different countries to carry out a worldwide earthquake competition in real time.